IN-STREAM TREATMENT OF MINE WATER USING CLAY
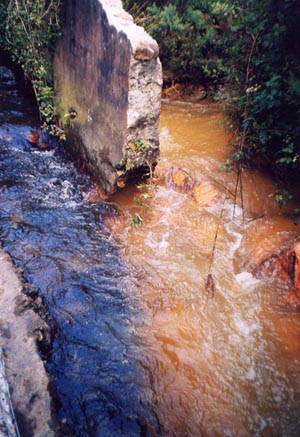
WATER FROM DOLCOATH ADIT STREAM (ON RIGHT) ENTERING THE RED RIVER (ON THE LEFT)
07-Nov-02
Dr Phil Smith, Aquatonics Ltd, Glenthorne, Searle Street, Crediton, Devon, EX17 2DB, UK. e-mail phil@aquatonics.com
1. INTRODUCTION
It has been estimated that in England and Wales there are at least 410 km of watercourses that are significantly affected by pollution caused by abandoned mine workings (National Rivers Authority, 1994). Of this total (which does not include many smaller watercourses) over half are in SW England, due to the long mining history in Cornwall and west Devon.
These abandoned mines cause a variety of pollution problems, including aesthetic ones due to the orange colour of many of the watercourses, caused by the deposit of ochre. There is no single organisation that is responsible for cleaning up these discharges (House of Commons, 1999). Any solutions to the problem of historical mine waters must be practical, robust and affordable. It would also be preferable to develop treatment systems that can cope with the diffuse nature of metal pollution on some catchments, with pollution at different locations due to adit drainage and mineral spoil heaps.
Aquatonics Ltd is carrying out a feasibility study into the use of dredged clays for removing metals from mine waters and industrial effluents. The feasibility study is funded under a DTI SMART award, administered by the Government Office of the South West.
Most of the research by Aquatonics Ltd has examined the use of clay in suspension to remove metals (Smith, 2000a, 2000b, 2001; Smith & Hook, 2002). In June 2002 laboratory trials at the University of Exeter showed that a 4 metre flume lined with glauconitic clay could remove copper highly effectively, without causing any problems with increased concentrations of suspended solids.
The ability of the clay flume to remove copper was tested at different concentrations of copper, using an outdoor recirculating system at Exeter University (report in progress). At the highest copper concentration tested (110 ppm or 110 mg/L) the first pass down the 4m flume removed 22 mg/L from 20 litres of synthetic effluent. This means that 440 mg of copper was removed in 10 minutes by 0.32 sq m of clay. This is equivalent to 137 mg Cu/sq m/minute. The rate of removal of copper by the clay flume fell with declining copper concentrations. Over the 4 hour test the mean removal rate of copper was 1.4 mg Cu/sq m/minute, due to the recirculating system continually feeding back lower copper concentrations. Over the last hour of the study the rate of removal of copper was 0.124 mg/sq m/minute (equivalent to 124 m g/sq m/minute).
The next stage in the design of an in-stream treatment using clays was to try out the clay flume on a mine-water effluent. This report provides data on metal removal at Dolcoath Adit, near Camborne. This site was suggested by the Environment Agency in Bodmin as a suitable location for the trials, but there are no plans to clean up the discharge from Dolcoath Adit. The aim of the trials were to ensure that laboratory results for metal removal could be replicated in the field.
The Dolcoath adit study has been useful in determining the rate of removal of copper per square metre of clay at much lower concentrations of copper (approximately 0.12 mg/L or 120 m g/L) than in the laboratory experiments. The Dolcoath Adit data showed a rate of removal of 102 m g/sq m/minute, quite similar to the rate of removal of 124 m g/sq m/minute at the end of the recirculating flume study described above.
2. STUDY SITE
The site suggested by the Environment Agency was the stream from Dolcoath Adit, near Camborne. This adit drains an underground network of mines that includes Dolcoath (which was closed in 1923) and South Crofty (closed in 1998).
Dolcoath Adit runs for a considerable distance underground, but on the surface it is only present for approximately 35 m before it joins the Red River at NGR SW 6485 4189. The cover photograph shows the water from Dolcoath Adit (on the right) joining the Red River. The orange colouration of the Dolcoath Adit Stream is due to the precipitation of ochre, which has the chemical formula FeOOH, but is also likely to contain a range of impurities.
Very little aquatic life can thrive in the Dolcoath Adit stream, but the macrophyte Potamogeton pectinatus was present at high densities in some parts.
It should be noted that the Environment Agency treat Dolcoath Adit as a discharge, rather than a watercourse. It does not, therefore have to meet Environmental Quality Standards (EQS). Any comments in this report regarding EQS values need to be understood in this context.
3. FLOWS
The Environment Agency monitor flows in the Dolcoath Adit stream. The mean flow over the period 1 March to 12 September was 119 L/sec (range 75 - 183 L/sec). On the two days of the field trials the flows were 106 and 105 L/sec. The data supplied by the EA shows a general decline in flows during the period March to September 2002.
4. METHODS
The sediment used was the same as in previous studies by Aquatonics Ltd. It is a mixture of clay (47%) and silt (50%). The remaining 3% was pieces of decomposing vegetation, mostly larger than 0.5 mm. Figure 1 shows the X-Ray Diffraction (XRD) analysis carried out by the British Geological Survey on the sample. This shows the presence of kaolinite, illite, glauconite and smectite. The metal content of the glauconitic sediment had previously been analysed by ICP-AES (Smith, 2000a). The iron content was 2.9%, other metals were measured at concentrations in the parts per million range (equivalent to mg/kg): magnesium 3860 ppm, calcium 1530 ppm, zinc 63 ppm, chromium 22 ppm, lead 18 ppm, copper 17 ppm and cadmium 4 ppm.
Dissolved oxygen (DO) was measured using a pHOX 62 DO meter. The DO meter was calibrated in air, with the air saturation value set to 100%. This is not an exact calibration method and DO results should be treated with some caution. The main aim was to find out if the flume caused any significant changes to the DO content of the water. DO concentrations were recorded as mg/L and percentage saturation.
Conductivity and pH was measured on site, within a few minutes of collecting the samples. The conductivity meter was a Hanna Dist 3, and the pH meter was a Hanna pHep. The pH and conductivity meters were calibrated daily using fresh standards.
The flume was made from three 4m sections of domestic guttering, with plastic joints every 4 metres. The clay bed was put in manually into each 4 m section. The total area of clay in the flume was 0.96 square metre (12m long by 0.08 m wide). The flume was suspended from wooden struts, about 12 cm above the stream surface and at the same gradient (Photos 1 & 2).
Before starting on the main series of experiments, samples were taken from the stream at the start and end of the flume to check that water quality (pH, conductivity, temperature and DO) did not change along this stretch of the stream. The results (Table 1) showed only minor changes in these parameters over the 12m length of stream.
Table 1. Water Quality in Dolcoath Adit stream at Start and Finish of Flume Section
4 July 2002 |
Time (BST) |
pH |
Conductivity (m
s/cm) |
Temperature (oC) |
DO
(mg/L) |
DO
(% saturation) |
Start |
14:50 |
5.8 |
719 |
16.7 |
6.90 |
72.0 |
Finish |
14:53 |
5.9 |
715 |
16.8 |
6.98 |
72.1 |
Water from the stream was then pumped into the flume using a domestic Salacia solar-powered water pump. This pump had an integral rechargeable battery for dark or cloudy periods that could, when fully charged, last up to 20 hours. The pump was powered by a 2.6 Watt thin film silicon solar panel. The stated pump capacity was 270 litres per hour.
Water samples were obtained in pairs on each occasion:
1. From the stream at the point where water was pumped into the flume, and
2. As it left the flume.
Sampling dates and times are shown in Table 2. Each pair of water samples was taken within a period of 10-15 minutes. The upstream sample was always taken first. The first part of each sample was used to fill a pre-labelled Environment Agency container for "Total" metals. Some of the remaining sample was then filtered using a 60 ml Plastipak ̉ disposable syringe fitted with a Minisart̉ 0.2 m m filter. This "Dissolved" fraction was put into a separate pre-labelled container provided by the Environment Agency.
Metals (As, Ca, Cd, Cr, Fe, K, Li, Na, Pb, and Zn) were analysed in both the total water sample (total metals) and in the filtered water samples (dissolved metals).
The flow through the flume was estimated by collecting water samples over time periods and weighing the water collected. The flow was calculated as litres per minute (Table 2). On the first day the mean flow through the flume was 3.41 L/minute. On the second day the mean flow was 2.72 L/min.
5. RESULTS AND DISCUSSION
Mean dissolved oxygen concentrations increased from 8.4 mg/L at the start of the flume to 10.0 mg/L at the end of the flume (Table 2). Some of this increase may have been due to the manner in which the samples were obtained, as there was a greater chance of entraining air in the water sample collected at the end of the flume. The pH increased very consistently, from 6.0 to 6.3. Temperature decreased very slightly, from 16.3 oC to 16.0 oC. Conductivity of the Dolcoath Adit stream was already high before treatment, and increased by about 10%, from a mean of 717 m S/cm to 781 m S/cm. This increase was probably due to the release of salts from the clay, which came from an estuarine site (Southampton Water). Increased conductivity from the use of marine or estuarine clays may be an issue in situations where long lengths of clay bed are required. We estimate that for most streams with a low initial conductivity the increase would have no adverse impacts for clay beds of less than 100 metres (additional 530 m S/cm). Above that distance there may be some minor effects on the benthic invertebrates and zooplankton that are able to colonise the stream.
A proportion of the ability of the glauconitic clay to remove metal ions is due to its cation exchange capacity (CEC) of 29.5 meq/100 g. Cation exchange is the process by which some of the metal ions on the clay are exchanged with more reactive metal ions in solution. In the case of clays the cations that are replaced include calcium, magnesium, sodium and potassium. Of these sodium and potassium were measured by the Environment Agency in the Dolcoath Adit study. Sodium (which is predominantly in dissolved form) increased by 28% and potassium by 33% (Table 3).
Concentrations of lead and chromium were below the detection limits and are not considered further. The remaining metals showed decreases ranging from 29% (dissolved copper) to 6-8% (dissolved zinc, nickel and arsenic). Dissolved iron showed a 17% reduction.
5.1 Arsenic
Dissolved arsenic before treatment was 4.3 ± 0.2 m g/L. After passage down the flume the mean dissolved arsenic concentration was 4.0 ± 0.2 m g/L. This represents a 5.8% decline in dissolved arsenic concentrations
Total arsenic before treatment was 47.6 ± 1.5 m g/L. After passage down the flume the mean total arsenic concentration was 44.6 ± 3.1 m g/L. This represents a 6.1% decline in total arsenic concentrations.
5.2 Cadmium
Dissolved cadmium before treatment was 2.76 ± 0.06 m g/L. After passage down the flume the mean dissolved cadmium concentration was 2.45 ± 0.5 m g/L. This represents an 11.0% decline in dissolved cadmium concentrations
Total cadmium before treatment was 2.68 ± 0.13 m g/L. After passage down the flume the mean total cadmium concentration was 2.50 ± 0.09 m g/L. This represents a 6.7% decline in total cadmium concentrations.
5.3 Copper
Dissolved copper before treatment was 118 ± 2.5 m g/L. After passage down the flume the mean dissolved copper concentration was 84 ± 7.1 m g/L. This represents a 29% decline in dissolved copper concentrations.
Total copper before treatment was 175 ± 11.8 m g/L. After treatment the total copper content was 148 ± 11.8 m g/L. This represents a 15% decline in total copper concentrations.
5.4 Iron
Dissolved iron before treatment was 2666 ± 155 m g/L. After passage down the flume the mean dissolved iron concentration was 2217 ± 121 m g/L. This represents a 17% decline in dissolved iron concentrations.
Total iron before treatment was 6273 ± 749 m g/L. After treatment the total iron content was 5834 ± 773 m g/L. This represents a 6.5% decline in total iron concentrations.
5.5 Nickel
Dissolved nickel before treatment was 56.6 ± 0.8 m g/L. After passage down the flume the mean dissolved nickel concentration was 52.1 ± 1.3 m g/L. This represents a 7.8% decline in dissolved nickel concentrations.
Total nickel before treatment was 57.9 ± 1.0 m g/L. After passage down the flume the mean total nickel concentration was 54.6 ± 1.8 m g/L. This represents a 5.7% decline in total nickel concentrations.
5.6 Zinc
Dissolved zinc before treatment was 1612 ± 37 m g/L. After passage down the flume the mean dissolved zinc concentration was 1492 ± 72m g/L. This represents a 7.4% decline in dissolved zinc concentrations.
Total zinc before treatment was 1685 ± 72 m g/L. After passage down the flume the mean total zinc concentration was 1571 ± 63 m g/L. This represents a 6.6 % decline in total zinc concentrations.
6. CALCULATING LENGTHS OF CLAY TREATMENT REQUIRED
The results from each metal are considered below, in relation to the UK Environmental Quality Standards (EQS) values (Table 4). The purpose of this section of the report is not to design a treatment method for Dolcoath Adit stream (which is a discharge rather than a watercourse) . Instead the aim is to take the data from the field trials as an example of a the quality that could occur in a stream affected by mine water and to show the length of stream bed that would be needed to provide treatment.
Several metals (arsenic, cadmium, chromium, lead & nickel) were below the relevant EQS for watercourses and are not considered further.
1. Copper
The EQS for copper for protection of freshwater life depends on the hardness of the stream water. The calcium in hard waters acts as a protective ion against copper toxicity, so higher concentrations of copper are allowed in hard waters than in soft waters. The Red River hardness is about 223 mg CaCO3 per litre (WRC, 1984). At this hardness the relevant EQS for dissolved copper (annual mean) is 10 m g/L and 95% of results should be less than 40 m g/L.
If the field data had come from a watercourse rather than a discharge (which does not need to meet an EQS) a copper removal of 92% (118-10/118) would have been required to achieve the EQS. If additional flume had been available, and the removal of dissolved copper was linear the EQS would have been achieved after a distance of:
(92%*12m)/29% = 38m
2. Iron
The EQS for dissolved iron for protection of freshwater life is 1000 ug/L as an annual average. This means that a iron removal of 62.5% (2666 - 1000/2666) would have been required (using field trial data) to achieve the EQS. If additional flume had been available, and the removal of dissolved iron was linear the EQS would have been achieved after a distance of:
(62.5%*12m)/17% = 44m
6.6 Zinc
The EQS value for total zinc to protect sensitive freshwater life (hardness range 100-250) is 75 m g/L as an annual average. For less sensitive species the corresponding EQS for total zinc is 250 m g/L. Using the field data the former would require 169m of clay flume, the latter would require 151m.
7. STREAM TREATMENT USING CLAY
For a stream affected by mine water discharges and with a similar chemical composition to Dolcoath adit stream the EQS values for As, Cd, Cr, Ni and Pb are already met before treatment. The length of clay bed needed to treat other metals to bring them down to the EQS is estimated to be:
Copper 38m
Iron 44m
Zinc 151m (for less sensitive species) or 169m (for more sensitive species)
It is clear that for this example the treatment system would have to be designed for zinc.
To achieve metal removals similar to those in the field trial would require the stream flow to be compartmentalised, so that a thin layer of polluted water passes over the clay. This is necessary to achieve intimate contact between the water and the clay. Aquatonics Ltd are actively pursuing this idea with the aim of developing a commercial system that can easily be installed in small metal-polluted streams.
7.1 Rate of Removal of Copper by Clay Flumes
The ability of the glauconitic clay flume to remove copper was tested at different concentrations of copper, using an outdoor recirculating system at Exeter University (report in progress). At the highest copper concentration tested (110 ppm or 110 mg/L) the first pass down the 4m flume removed 22 mg/L from 20 litres of synthetic effluent. This means that 440 mg of copper was removed in 10 minutes by 0.32 sq m of clay. This is equivalent to 137 mg Cu/sq m/minute. The rate of removal of copper by the clay flume falls with declining copper concentrations. Over the 4 hour test the mean removal rate of copper was 1.4 mg Cu/sq m/minute, due to the recirculating system continually feeding back lower copper concentrations. Over the last hour of the study the rate of removal of copper was 0.124 mg/sq m/minute (equivalent to 124 ug/sq m/minute).
The Dolcoath adit study has been useful in determining the rate of removal of copper per square metre of clay at much lower concentrations of copper (approximately 0.12 mg/L or 120 m g/L). The Dolcoath Adit data showed a rate of removal of 106 m g/sq m/minute, quite similar to the rate of removal of 124 m g/sq m/minute at the end of the recirculating flume study described above.
This information can be used to design treatment systems for metal-polluted streams, and industrial effluents, especially if copper is the main metal of concern. The two main options for treatment with clays are clay slurries and clay beds or flumes. These are considered in Section 7. Clay beds could be described as "in-stream treatment" and would not require the polluted water to be pumped, or large areas of land to be set aside for treatment purposes. It may, however, be necessary to have a settling pond downstream of each section of treatment.
8. CALCULATING THE AMOUNT OF CLAY REQUIRED TO TREAT A CONTAMINATED STREAM
A visit by Aquatonics Ltd to the River Lynher tributary in June 2000 showed that there were contaminated tributaries that were potentially suitable for treatment using clays. Data for metal concentrations and flows in tributaries of the Rivers Lynher are contained in a PhD thesis (Burton, 1998). The most copper polluted site in her study was in the upper reaches of the Darley stream. Mean dissolved copper concentrations fell from the highest point on the Darley (D5, mean 766m g/L) to the lowest point sampled (D1, mean 128 m g/L). Maximum copper concentrations fell from 928 m g/L at D5 to 247 m g/L at the lowest site, D1.
Mean pH increased from 6.2 at site D5 to 6.6 at site D1. Maximum flow at D5 was 39 L/s, this increased to 261 L/s at D1. The total quantity of dissolved copper transported during high flows was 1.94 g/minute at D5 (autumn flows) and 3.87 g/minute at D1 (winter flows). If mean copper concentrations are multiplied by mean flows the rate of copper transported is 1.15 g/minute at D5 and 1.45 g/minute at D1. These figures suggests that there is either no natural treatment within Darley stream or that it is offset by other inputs of copper.
The data for Darley stream have been used by Aquatonics Ltd to calculate the amount of treatment required using either clay beds or clay in suspension.
8.1 Treatment using clay beds
Darley Stream has a mean copper concentration at D5 of 766 m g/L, about 6.5 times that in Dolcoath Adit. A higher rate of removal of copper per square metre of clay would be expected in Darley Stream compared to Dolcoath Adit. The maximum flow rate at site D5 was 39 l/s. If a copper removal rate of 200 m g/sq m/minute can be achieved by a clay bed (which is at the lower end of the removal rates we have achieved to date) the total area required for site D5 would be 8962 square metres. If this area could be provided as a shallow pond it would be 95m x 95m (approximately 0.9 ha). If it had to be provided within the existing dimensions of the stream (which is about 2m wide) the total length of clay bed required would be 4481m, which is longer than the total stream length (estimated at 3000m). If the flow can be compartmentalised so that there are 10 horizontal layers of treatment the total length required falls to 448m.
8.2 Treatment using clay in suspension
Smaller areas of treatment would be required if clay suspensions could be used and the clay trapped in settling ponds. Assuming three settling ponds down the stream with an average area of 10m x 10m the total area required would be 300 square metres. In addition there would be a small area for dosing the clay into the stream above each settling pond. The details of the design could only be determined after further field trials, but it is possible to calculate the amount of clay required.
In the laboratory trials it was possible to obtain 83000 mg/kg (8.3%) copper on the clay, when very low clay concentrations were used. This was achieved with a copper concentration of 50 mg/L and a mixing time of 5 minutes. Lower concentrations of copper on the clay would be expected in Darley Stream, due to the lower copper concentration in the water (mean 0.766 mg/L). This would be offset to some extent by the much longer contact time, which could be a few hours. As an estimate it may be possible to get 1% copper on the clay. The amount of dissolved copper transported by Darley Stream at site D5 is estimated to be 600 kg/annum. To remove all of this onto the clay would require 60,000 kg of clay to be dosed in each year. This is equivalent to 0.0019 kg/sec, or 1.90 g/sec, or 1900 mg/s. The mean discharge at D5 was 25l/s (Burton, 1998). If none of the clay added to the stream settled out the additional suspended solids would be 1900/25 = 76 mg/L. At least 67% of this material would need to be removed by settling to meet suspended solid criteria for salmonid rivers if flow remained the same down the stream. However, flow increases down Darley stream, with a mean discharge of 189 L/s at D1. At the point where the Darley joins the River Lynher the additional suspended solids concentration in Darley stream is predicted to be 10.1 mg/L, which may be acceptable to the Environment Agency. This water would receive further dilution on reaching the River Lynher.
The cost of dosing 60 tonnes of clay per annum need to be estimated, but would not be expected to be very high. The settling ponds would need to be cleaned, probably on a 1-2 year cycle. The contaminated sediment could either be disposed of at a landfill site, or in the long term it may be possible to use it for brick production, as happens in Germany. It is also possible that the copper could be leached from the clay by acid treatment and the clay could then be re-used. The copper liquor could be electrolysed to produce metallic copper.
The above calculations assume that all of the copper is present at site D5, but an estimated additional 160 kg of copper a year is transported at site D1 (approximately 762 kg of dissolved copper per annum). This would require an additional 16,000 kg of clay to be dosed annually somewhere between D5 and D1. At D1 the total additional suspended solid concentration is estimated to be 12.8 mg/L (10.1 mg/L from main dosing plus 2.7 mg/L from additional dosing).
9. THE ADVANTAGES OF IN-STREAM TREATMENT
In-stream treatment has several advantages:
simplicity - very little to go wrong
robustness - unlikely to have down-time
flexibility 1 - could be installed at many different sites down a stream to treat diffuse discharges
flexibility 2 - as mine water quality improves the length of stream treated can easily be reduced. Conversely extra units could be added if EQS values are tightened.
low capital costs
low maintenance costs
potential for community involvement
The main disadvantage is that a considerable proportion of the total length of stream may need to have treatment, and this is likely to be visible. However, compared to the very poor aesthetic quality of most streams that are adversely affected by mine waters this should not be a problem.
10. REFERENCES
Burton, SM (1998). Evaluation of stream meiofauna as a monitor of trace metal contamination. PhD Thesis, Department of Biological Sciences, University of Plymouth.
House of Commons (1999). Treatment of Acid Mine Drainage. House of Commons Research Paper 99/10.
National Rivers Authority (1994). Abandoned Mines and the Environment. NRA Water Quality Series No 14, March 1994.
Smith, PRJ (2000a). The Use of a Glauconitic Clay to Remove Metals from Solution. Aquatonics Ltd.
Smith, PRJ (2000b). Use of a Glauconitic Clay to Remove Copper from Effluents Produced During Manufacture of Printed Circuit Boards. Aquatonics Ltd.
Smith, PRJ (2001).Trials of a Prototype Plant using Clay Slurry to Remove Metals from a Mine Effluent. Aquatonics Ltd.
Smith, PRJ & Hook, M (2002). Comparison of Different Clay Slurries for Removing Copper from Solution. Aquatonics Ltd
WRC (1984). Proposed environmental Quality Standards for List II Substances in Water. Copper. WRC Technical Report TR 210.
11. ACKNOWLEDGEMENTS
Susan Burton, data from her study sites on tributaries of the River Lynher and field visit to the Darley Stream.
Myles Hook, assistance with field trials.
Peter Long & Julian Greaves, Environment Agency, Bodmin. Advice on a suitable site, arrangements for metal analyses and provision of flow data for Dolcoath Adit.
John Norton, freelance botanist, identification of the plant Potamogeton pectinatus from Dolcoath Adit stream.
David Stone, Baseresult Holdings Ltd.
Paul Tiltman & Bill Streets, Government Office of the South West.
TABLE 2. SAMPLING DETAILS AND ANALYSES ON SITE
TABLE 3. METAL DATA
TABLE 4. EQS VALUES FOR METALS
METAL
(only those above detection limit in this study are listed) |
HARDNESS
(mg/L CaCO3) |
EQS TO PROTECT FRESHWATER LIFE |
Arsenic |
|
Dissolved arsenic: 50 m
g/L as an annual mean |
Cadmium |
|
Total cadmium : 5 m
g/L as an annual mean |
Copper |
Hardness
0-50
50-100
100-250
>250 |
Dissolved copper as an annual mean
1 m
g/L
6 m
g/L
10m
g/L
28 m
g/L |
Iron |
|
Dissolved iron: 1000 ug/L as an annual mean |
Nickel |
0-50
50-100
100-200
>200 |
Dissolved nickel: as an annual mean
50 m
g/L
100 m
g/L
150 m
g/L
200 m
g/L |
Zinc |
0-50
50-100
100 -250
>250
0-50
50-100
100-250
>250 |
Total zinc (sensitive freshwater life) as annual mean:
8 m
g/L
50 m
g/L
75 m
g/L
125 m
g/L
Total zinc (less sensitive species) as annual mean:
75 m
g/L
175 m
g/L
250 m
g/L
500 m
g/L |